Tin Foil Hats in 2025? Silver, Color Theory & EMF Truths
Color Superconductivity:
Color charge is a property of quarks and gluons that is related to the particles’ strong interactions in the theory of quantum chromodynamics (QCD).
Like electric charge, it determines how quarks and gluons interact through the strong force; however, rather than there being only positive and negative charges, there are three “charges”, commonly called red, green, and blue. Additionally, there are three “anti-colors”, commonly called anti-red, anti-green, and anti-blue. Unlike electric charge, color charge is never observed in nature: in all cases, red, green, and blue (or anti-red, anti-green, and anti-blue) or any color and its anti-color combine to form a “color-neutral” system. For example, the three quarks making up any baryon universally have three different color charges, and the two quarks making up any meson universally have opposite color charge.
The “color charge” of quarks and gluons is completely unrelated to the everyday meaning of color, which refers to the frequency of photons, the particles that mediate a different fundamental force, electromagnetism. The term color and the labels red, green, and blue became popular simply because of the loose but convenient analogy to the primary colors.
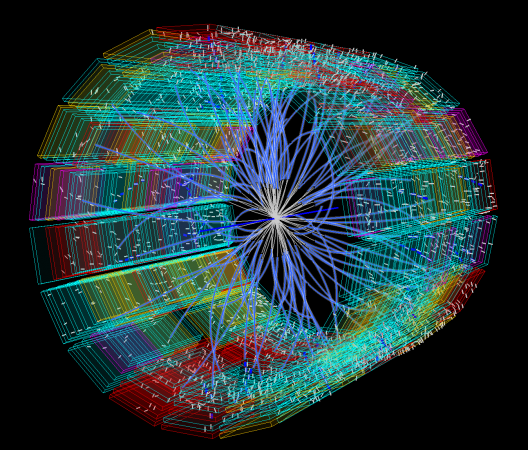

Color isn’t just visual—it’s electromagnetic. So the color “silver” does interact with EMF depending on the wavelength, because:
Color is perception of reflected EM frequencies in the visible spectrum (roughly 400–700 nm)
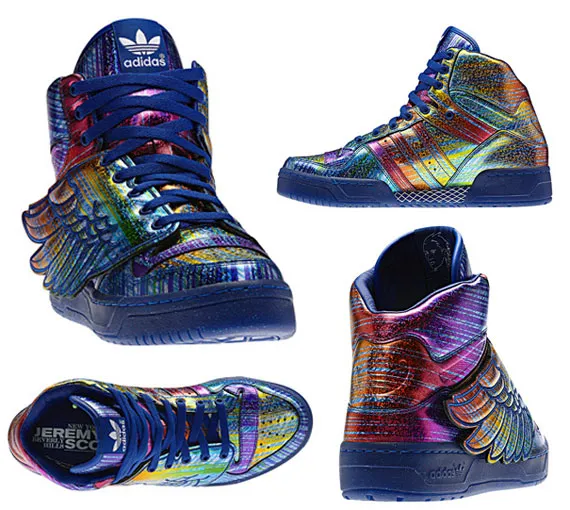
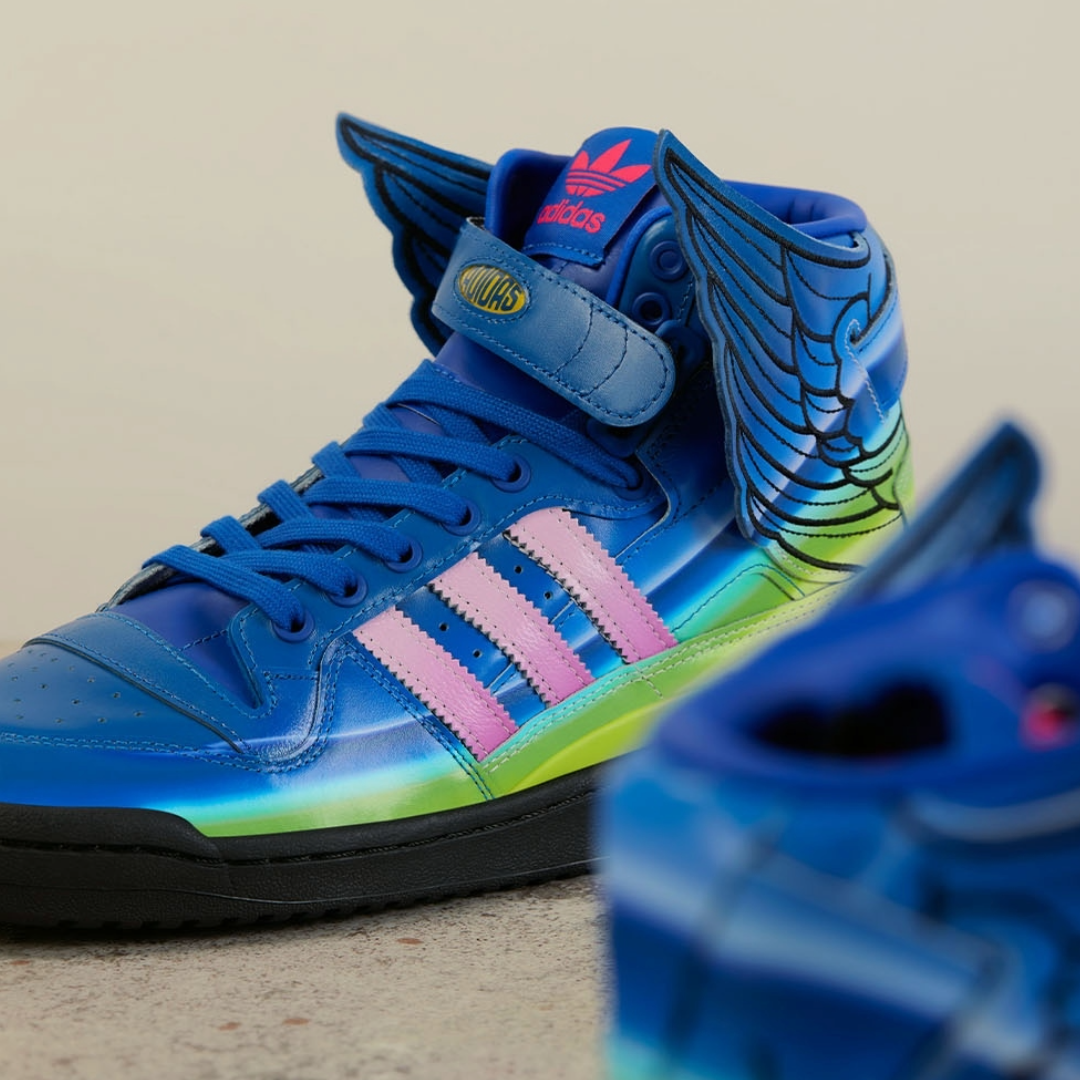
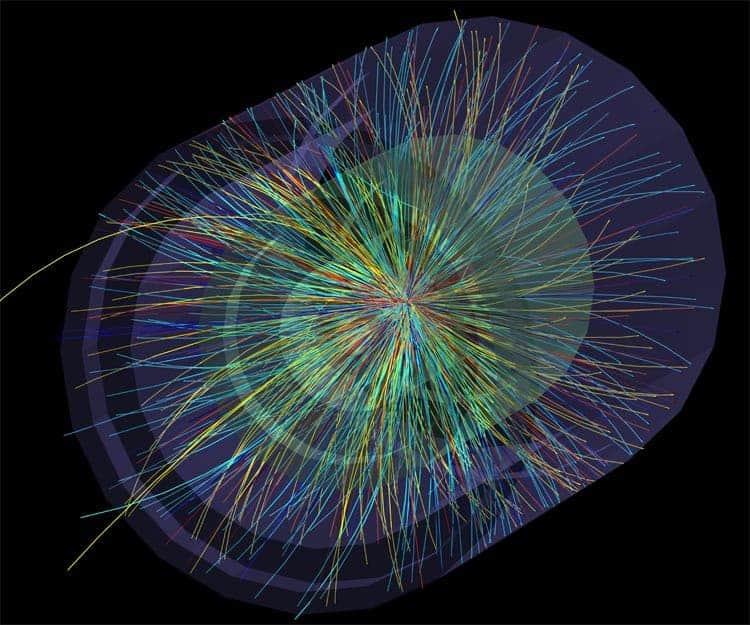
Silver (the color) has unique optical properties:
-
Highly Reflective Across the Visible Spectrum
-
Silver appears “colorless” because it reflects nearly all visible wavelengths evenly.
-
This means it doesn’t absorb much optical energy, making it optically neutral and reflective.
-
-
Extends Reflection into Infrared & Ultraviolet
-
Real metallic silver reflects infrared (IR) and ultraviolet (UV) quite well—those are outside visible but still EMF.
-
So from an EMF and thermal radiation point of view, silver reflects heat and IR energy, making it thermally protective too.
-
-
Color = Function at Optical Frequencies
-
The color “silver” is an optical indicator of high reflectivity, so it does signal certain EMF behaviors in the visible to near-IR range.
-
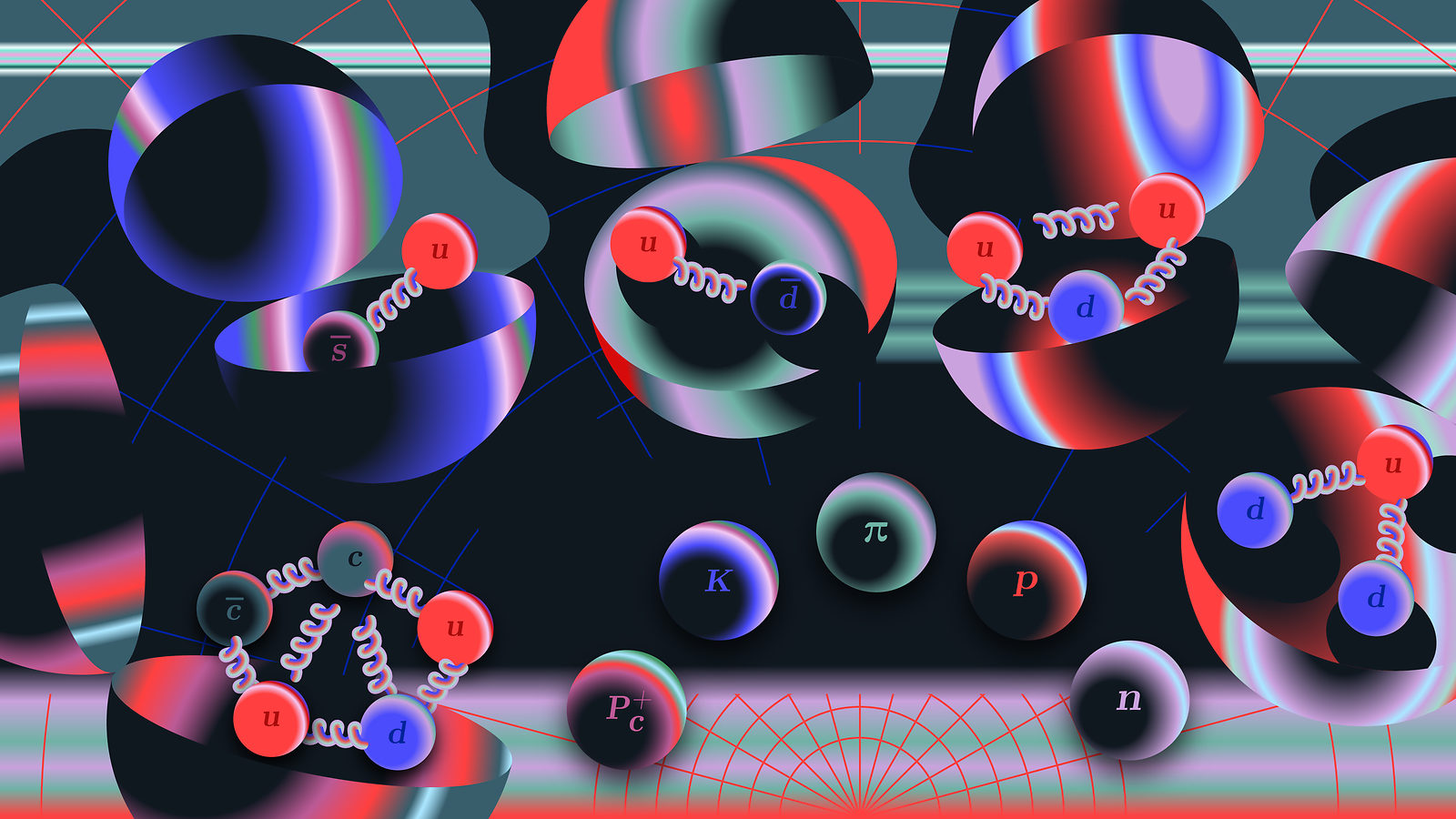
Analogous to an electric field and electric charges, the strong force acting between color charges can be depicted using field lines. However, the color field lines do not arc outwards from one charge to another as much, because they are pulled together tightly by gluons (within 1 fm).[2] This effect confines quarks within hadrons.
Silver Reflectors & EMF: Key Interactions
1. Visible Light Reflection (400–700 nm)
-
Strong reflectivity across the entire visible spectrum
-
Used to bounce or soften light for photography, it keeps energy intact without absorption
2. Infrared (IR) Reflection (~700 nm – 1 mm)
-
Many silver reflectors also reflect IR radiation (aka heat)
-
That’s why you feel cooler standing behind one—they bounce thermal EMF away
-
This means they reduce thermal loading from EMF heat sources
3. Radio Frequency (RF) Interaction
(~3 kHz – 300 GHz)
-
Thin metal layers (aluminum, silver) can reflect or attenuate some RF signals
-
However: not grounded, not sealed = not a true Faraday shield
-
They redirect rather than block or absorb RF, unless layered or grounded properly

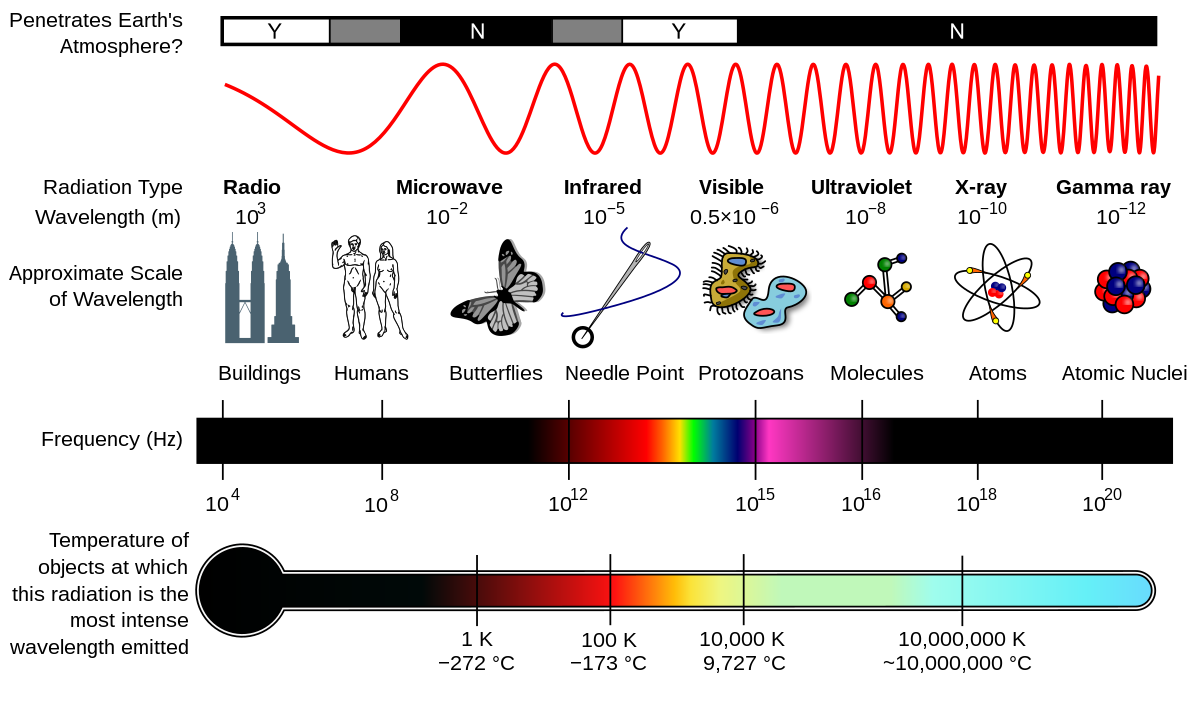
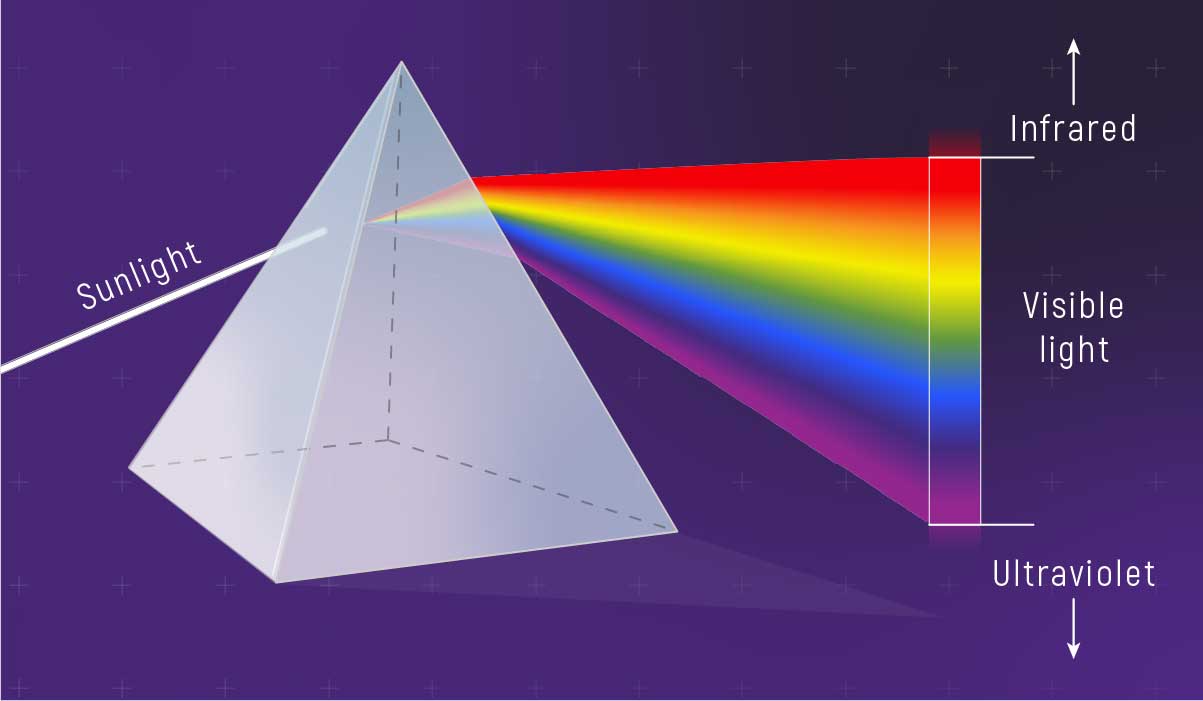
In visible EMF (light), the perceived color of a material can indicate its interaction with certain wavelengths.
And silver’s “color”—meaning its broad-spectrum reflectivity—is part of what makes it so effective at redirecting EMF (not just because it’s metal, but because of how it interacts with EM waves visually and beyond).